(1) Institut für Geowissenschaften, Universität Potsdam, Germany (2) Laboratoire de Géologie, ENS Paris-UMR 8538, France (3) Geologisches Institut, Universität Basel, Switzerland (4) Department für Lithosphärenforschung, Universtät Wien, Austria (5) Geological Survey, Vienna, Austria (6) Institute für Geologie, Universität Bern, Switzerland (7) Dipartimento di Scienze della Terra, Università degli Studi di Torino, Italy Citation of this article:
Bousquet R., Oberhänsli R., Goffé B., Wiederkehr M., Koller F., Schmid S. M., Schuster R., Engi M., Berger A. & Martinotti G., 2008 Metamorphism of metasediments in the scale of an orogen: A key to the Tertiary geodynamic evolution of the Alps. in Tectonic Aspects of the Alpine-Dinaride-Carpathian System Eds edited by Siegesmund, S., Fügenschuh, B. & Froitzheim, N. Geological Society, London, Special Publications, 298, 393-412
Major discoveries in metamorphic petrology, as well as other geological disciplines, have been made in the Alps. The regional distribution of late Cretaceous-Tertiary metamorphic conditions, documented in post-Hercynian metasediments across the entire Alpine belt from Corsica-Tuscany in the West to Vienna in the East, is presented in this article. In view of the uneven distribution of information, we concentrate on type and grade of metamorphism; and we elected to distinguish between metamorphic paths where either pressure and temperature peaked simultaneously, or where the maximum temperature was reached at lower pressures, after a significant temperature increase on the decompression path.
The results show which types of process caused the main metamorphic imprint: a subduction process in the Western Alps, a collision process in the Central Alps, and complex metamorphic structures in the Eastern Alps, owing to a complex geodynamic and metamorphic history involving the succession of the two types of processes. The Western Alps clearly show a relatively simple picture, with an internal (high-pressure dominated) part thrust over an external greenschist to low-grade domain, although both metamorphic domains are structurally very complex. Such a metamorphic pattern is generally produced by subduction followed by exhumation along a cool decompression path. In contrast, the Central Alps document conditions typical of subduction (and partial accretion), followed by an intensely evolved collision process, often resulting in a heating event during the decompression path of the early-subducted units. Subduction-related relics and (collisional/decompressional) heating phenomena in different tectonic edifices characterize the Tertiary evolution of the Eastern Alps. The Tuscan and Corsica terrains show two different kinds of evolution, with Corsica resembling the Western Alps, whereas the metamorphic history in the Tuscan domain is complex owing to the late evolution of the Apennines. This study confirms that careful analysis of the metamorphic evolution of metasediments at the scale of an entire orogen may change the geodynamic interpretation of mountain belts.
After a more than a century of investigations, the Alps still represent an outstanding natural laboratory for the study of geodynamic processes linked to the evolution of mountain belts in general. The integration of regional geology and metamorphic evolution provides highly needed constraints for increasingly complex quantitative models (e.g. Escher and Beaumont 1996; Henry et al. 1997; Pfiffner et al. 2000).
Major discoveries in metamorphic petrology, as well as other geological disciplines, have been and are still made in the Alps. For example eclogites were described for the fist time in the Eastern Alps (Koralpe, Saualpe massifs) by Haüy (1822). More recently the discovery of coesite in the Dora Maira unit (Chopin 1984, 1987) proved that continental crust went into subduction, contrary to a still widely held opinion, and returned from great depths. Many others, occasionally less spectacular yet important, petrological discoveries were also made in the Alps. For instance, studies of metapelites in the Alps starting at the beginning of the 1970′s, revealed a specific mineralogy reflecting high-pressure conditions. The most emblematic minerals found in such rocks are ferro- and magnesiocarpholites (Goffé et al. 1973). Besides such discoveries many holistic attempts have been made to assess the dynamics of this orogen. Niggli and Niggli (1965) applied Barrow′s concept to the Central Alps and presented a mineral distribution map with mineral isogrades reflecting a Lepontine high-temperature event. Zwart (1973) and Zwart et al. (1978) compiled mineral distributions at the scale of the orogen, using the facies concept and available age data in the Alps and elsewhere.
Based on such mineralogical work, Ernst (1971) was able to use the plate tectonic concept for proposing a first modern model for the evolution of the Alps. Such ideas developed further on the base of work, such as that of Dal Piaz et al. (1972), Dal Piaz (1974a, b) and Hunziker (1974), just to name a few. Frey (1969), as well as Trommsdorff (1966), started to investigate metamorphism in isochemical systems provided by shales and siliceous carbonates, respectively. This allowed for quantitatively constraining the Cenozoic temperature evolution in the Central Alps. Frey et al. (1999) compiled all available information on the peak temperature distribution, and used the occurrence of eclogites to display the dynamics of the Alpine evolution. Previous works of this kind led to the compilation of a new map showing the metamorphic structure of the Alps (Oberhänsli et al. 2004). This new map was also based on (1) new tectonic concepts and maps (Schmid et al, 2004), (2) a wealth of new radiogenic age data (for references see for example Handy and Oberhänsli, 2004, and Berger and Bousquet, this volume), and (3) an extension of the facies concept based on mafic to metapelitic rock-compositions.
In the Alps many areas are devoid of index minerals classically observed in mafic and quartzo-feldspathic rocks systems, allowing a direct comparison to be made. Petrological investigation on metasediments greatly helps to constrain geodynamic evolution of such areas (see Bousquet 2007). One tool to understand such problems better at the orogen scale are maps (Niggli and Niggli 1965; Niggli 1970; Frey et al. 1999, Oberhänsli et al. 2004). This study combines these different informations: presenting metamorphism in maps and combining this with metamorphic evolution data. This provides insights in the geodynamics of metasediments inside the orogen. The metamorphism of metasediments can be subdivided into general geodynamic groups: (1) pressure-dominated metamorphism; (2) temperature-dominated metamorphism at intermediate pressures (Barrovian metamorphism), which is often referenced in the literature as HT metamorphism; and (3) contact metamorphic aureoles which are temperature-dominated metamorphism at low-pressures. The latter type will be excluded of this contribution because it is only of minor importance in the Alps.
This paper reviews existing data and presents ongoing work in an attempt to integrate metamorphic studies and late Cretaceous- Tertiary geodynamic concepts in the Alps. We will illustrate how mineral data obtained from meta-sediments may constrain the geodynamic evolution of mountain belts in general.
In contrast to mafic complexes or meta-igneous rocks, metasediments commonly crop out continuously over very large areas in many mountain belts, such as the Alps (Fig. 1). Since these metasediments cover large areas this allows to simultaneously observe their structural and metamorphic evolution, and thus to decipher the geodynamic frame. However, since Barrow (1893, 1912) and Eskola (1929) the definition of metamorphic facies, as well as petrographic work on metamorphic rocks, mainly focused on mafic systems (Evans 1990; Frey et al. 1991; Carswell 1990).
click on the picture to zoom or click here to download the picture
Figure 1: Structural units of the Alps involved and metamorphosed during the late Cretaceous - Tertiary orogeny from Vienna (Austria) to Corsica and Tuscany. The distribution of metasediments, ophiolites and basement rocks is not homogeneous over the whole alpine edifice. In the west, in Tuscany, Corsica and Western Alps, the belt is mainly built of post Hercynian metasediments from oceanic (mainly Piemont-Liguria) or continental (Briançonnais cover and European plateform) origin. Several ophiolitic complexes are associated with oceanic sediments (Zermatt, Lanzo, Viso, Voltri). Scarce fragment of basement rocks occur as structural domes (Monte Rosa, Gran Paradiso, Ambin, Dora Maira, Tenda) in the internal parts or are located at the external border of the belt (Mont Blanc, Belledonne, Pelvoux, Argentera). From the Simplon line in the Central Alps to the Eastern Alps mainly basement rocks have been imbricated into the orogenic pile (Lepontine dome, Tauern Window) except for the Engadine window. Upper Austroalpine units are not detailed on the map. Map modified after Froitzheim et al. (1996) and Schmid et al. (2004) with additional data from Bigi et al. (1990) and Koller and Höck (1987) |
Detailed studies on pelitic systems (Yardley 1989; Koons and Thompson 1985; Spear 1993; McDade and Harley, 2001) are only available for medium to high temperature metamorphic conditions. Metamorphic studies addressing low temperature conditions extended methods taken from studies on diagenesis or anchimetamorphism, such as illite cristallinity, vitrinite reflectance or clay mineralogy (Frey and Robinson 1999) which lack good possibilities of pressure and temperature calibration. Spectacular improvements on the knowledge of mountain belt evolution based on the study of metasediments could only be made starting with the discovery of coesite in metasediments (Chopin 1984) and other work on Ultra High Pressure rock systems (UHP) in general (Coleman and Wang 1995; Chopin and Sobolev 1995; Massonne and O′Brien, 2003).
Petrogenetic grids
Classical index minerals, such as pumpellyite, glaucophane or jadeite, observed in mafic and quartzo-feldspathic rocks systems, are unfortunately rarely observed in Alpine metasediments. Nevertheless, metasediments have highly variable chemical and mineralogical compositions that represent an important geothermobarometric potential. Fig. 2 shows petrogenetic grids for the KFMASH and CFMASH subsystems that integrate field observations, experimental data and thermodynamic modelling using an internally consistent database. This new kind of compilations covers a large P-T space, extending from low to high pressure (0-2 GPa) as well as from low to high temperature (200-800°C).
Figure 2: Petrogenetic grids for metapelites for a temperature range from 200 to 800°C. a) in the KFMASH (K2O-FeO-MgO-Al2O3-SiO2-H2O) system, the grid is strongly temperature-controlled. The appearance of assemblages, from (Fe, Mg)-carpholite assemblage at HP or from chlorite-pyrophylite assemblage delimits the low-temperature domain from the middle temperature one at around 400°C. The exact temperature limit depends on rock and mineral chemistry. At higher temperature conditions, the breakdown of chloritoid into garnet or staurolite indicates the transition towards high-temperature domains between 500 and 600°C depending on pressure conditions as well as on rock and mineral chemistry. b) In the CFMASH (CaO-FeO-MgO-Al2O3-SiO2-H2O) system, the temperature control is less important. While under LT conditions, lawsonite is the main stable mineral, sometimes coexisting with (Fe, Mg)-carpholite, at middle and HT conditions, margarite and staurolite stability fields are pressure-dependent. We note a large cordierite-stability field in the CFMASH system. Diagrams drawn from field experience and theoretical studies after Spear and Cheney (1989), Spear and Wang (1991), Vidal et al. (1992), Oberhänsli et al. (1995), Bousquet et al. (2002), Proyer (2003), Wei et al. (2004), Wei and Powell (2004), Wei and Holland (2003), Chatterjee (1976), Frey (1972), Zeh (2001), Pattinson et al. (2002), McDade and Harley (2001), Kohn and Spear (1993), Hébert and Ballèvre (1993) as well as own calculation using the Theriak-Domino software (De Capitani and Brown 1987, De Capitani (1994) using Berman database (1988) completed by recent thermodynamic data: Mg-chloritoid data of B. Patrick (listed in Goffé and Bousquet 1997), Fe-chloritoid data of Vidal et al. (1994), chlorite data of Vidal et al. (2001), and alumino-celadonite data from Massonne and Szpurka (1997). Mineral abbreviations are from Bucher and Frey (1994) except for (Fe, Mg)-carpholite (Car) |
Mineral assemblage containing ferro-magnesio-carpholite with phengite, chlorite and quartz is one of the most emblematic mineral assemblage of metasediments in the KFMASH system (De Roever 1951, Goffé et al. 1973; Chopin and Schreyer 1983; Goffé and Chopin 1986, Rimmelé et al. 2003). It is encountered in various rock types, such as aluminium-rich metapelites, quartzites, marbles and albite-free pelitic schists in which it is abundant, often in veins. Textural and mineralogical observations in these rocks reveal that at low temperatures the main equilibrium reactions of ferro- and magnesio-carpholite involve quartz, kaolinite, pyrophyllite, kyanite, chlorite, chloritoid and phengite (Fig. 2a). At high temperatures large PT-fields are dominated by assemblage containing staurolite, biotite and garnet (Spear and Cheney 1989). Fe-Mg variations in mineral composition, as a function of P and T (Goffé 1982; Spear and Selverstone 1983; Vidal et al. 1992; Theye et al. l992), as well as Si isopleths in phengite (Massone and Schreyer 1987; Oberhänsli et al. 1995; Massone and Szpurka 1997; Bousquet et al. 2002) allow for relatively precise P-T estimates (Vidal et al. 2001; Parra et al. 2002a, b; Rimmelé et al. 2005) for some metapelitic compositionsIn the CFMASH system, for comparison, there is less resolution at low temperature conditions. In carbonaceous systems the stability field of the index mineral lawsonite covers the whole low-grade space, including the stability field of carpholite and the aragonite-calcite transition. While the staurolite field is substantially smaller in the CFMASH system, as compared to the KFMASH system, margarite and zoisite are characteristic of medium P and T conditions.
Oberhänsli et al. (2004) proposed a new type of metamorphic facies grid better integrating field observations into models of the geodynamic. This facies grid also taken into account the importance of metasediments, which is less clear in traditional grids. The proposed grid also involved more subdivisions, which are based on the understanding of the metasediments. Based on these compilations a revised version of this tool is presented in Figure 3, and it will be used in this paper.
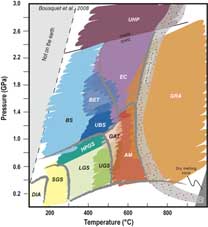 Figure 3: Metamorphic facies diagram for metapelites and metabasites (modified after Oberhänsli et al. 2004). This diagram has been used for figures 6 and 7. For abbreviations see Table 2. This diagram is in good agreement with previous published facies diagram (e.g. Yardley, 1989; Spear, 1993; Bousquet et al., 1997) |
Ambiguity of some mineral assemblages in the Alps
While some parageneses unambiguously grow only within a certain geodynamic context (Table 1a) other mineral assemblages commonly occur over a large field of P-T conditions and may evolve in diverse geodynamic contexts. Examples of unambiguous mineral assemblages containing lawsonite and carpholite only form under low-temperature/high-pressure conditions, typical for subduction processes; mineral assemblages containing staurolite and andalusite are typical high-temperature phases occurring during collision processes (i.e. Barrovian-type metamorphism).
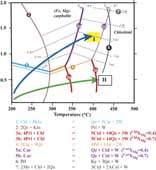 Figure 4: Ambiguity of chloritoid-quartz as index assemblage. Depending on the P-T path, chloritoid-quartz can be formed either by breakdown of (Fe, Mg)-carpholite at HP conditions (reaction 5) or by breakdown of pyrophyllite at LP conditions (reaction 3). Chloritoid-quartz assemblage alone cannot be used as index for pressure conditions. Its significance depends on the mineral reaction and on the associated minerals. |
On the other hand recurrent minerals such as chloritoid, zoisite, kyanite and garnet may form at different P-T conditions. This hampers their use for an interpretation of the geodynamic setting. Chloritoid-phengite assemblage, for example, can be produced in different geodynamic settings. Moreover it may occur in tectonic units in close spacial juxtaposition although these units formed in different geodynamic scenarios (Oberhänsli et al. 2003). Figure 4 clearly illustrates that two P-T paths may lead to different mineral reactions that produce mineral assemblages chloritoid-phengite-chlorite. The P-T path along a cold geotherm leads to the formation of chloritoid-phengite-chlorite as a result of the breakdown of Fe-Mg carpholite (Chopin & Schreyer 1983) while under higher geothermal gradients this assemblage can also form by the breakdown of pyrophyllite (Frey and Wieland 1975).
The high-pressure alumosilicate polymorph, kyanite, is particularly difficult to interpret as an indicator of geodynamic processes. It may occur in UHP associations such as the Dora Maira unit, as well as in temperature-dominated areas such as the Lepontine dome. It indicates a subduction-type low geothermal gradient in the first, but a high collision-related geotherm in the latter case.
Ambiguity of some metamorphic facies
Assignment of a metamorphic rock to a metamorphic facies is based on its mineralogy. In the best case a rock might undergo a simple metamorphic evolution in a distinct geodynamic setting, leading to a peak metamorphic paragenesis (simultaneous P and T peaks) possibly followed by later retrogression (Fig. 5a). However, a metamorphic pressure-peak related to one geodynamical scenario may also have been overprinted by a thermal peak that resulted from a second and different geodynamic scenario (Fig. 5b). Hence, in such a case metamorphic facies is ambiguous in that it is difficult to distinguish between continuous and discontinuous evolutions. It is only the exact shape of the P-T path, details that are often difficult to constrain, which is specific for a complex geodynamic evolution. For example, the significance of the amphibolite facies mineralogy is ambiguous. It may either represent a single path that entirely formed in a collision setting; alternatively it may merely represent an exhumation stage that formed during ongoing subduction and before final collision (Fig. 5a). In the case of dual peak paths details regarding amphibolite facies overprint are crucial for better understanding exhumation processes in general and details of the transition from subduction to collision in particular.
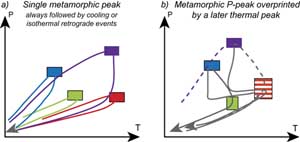 Figure 5: Ambiguity of some metamorphic facies. a) a rock undergoes a simple metamorphic evolution in a distinct geodynamic setting, leading to only one peak metamorphic paragenesis (simultaneous P and T peaks) followed by later retrogression. b) In some cases certain metamorphic PT paths show distinct pressure and temperature peaks. The pressure-peak can be related to one geodynamical scenario and it may have been overprinted by a thermal peak that resulted from a second and different geodynamic scenario. |
The metamorphic data in a geodynamic context
The above described importance of metasediments and the presented tool of a facies grid with their characterisitc mineral assemblages can be well used in the Alps. The Alps are well suited for such a compilation, because they are a relatively small and well-investigated orogen (e.g. Frey et al. 1974 1980; Goffé and Chopin 1986; Roure et al. 1990; Dal Piaz, 2001; Oberhänsli et al. 2004; Schmid et al. 2004). The Alps are developed by subduction of two different oceans followed by collision between two main continents (Adria and European). The relics of the oceans (Piemont-Liguria, Valais) and the separating microcontinent (Briançonnais) are still existing and are now sandwiched between of Adria and Europe. The paleogeographic (tectonic) overview of the present day situation is principally inspired from Schmid et al. (2004) and Bigi et al. (1990) and can so combined with metamorphic information of the different units. This approach will be presented below
All the way from the Adriatic margin preserved in the lower Austroalpine nappes to Europe-derived nappes the tectonic units (i.e. Piemont-Liguria, Briançonnais, Valais) contain metasediments that recorded the late Cretaceous-Cenozoic subduction history. The minerals indicating subduction-related processes are listed in Table 2 and their distribution are shown in Figure 6.
click on the picture to zoom or click here to download the picture
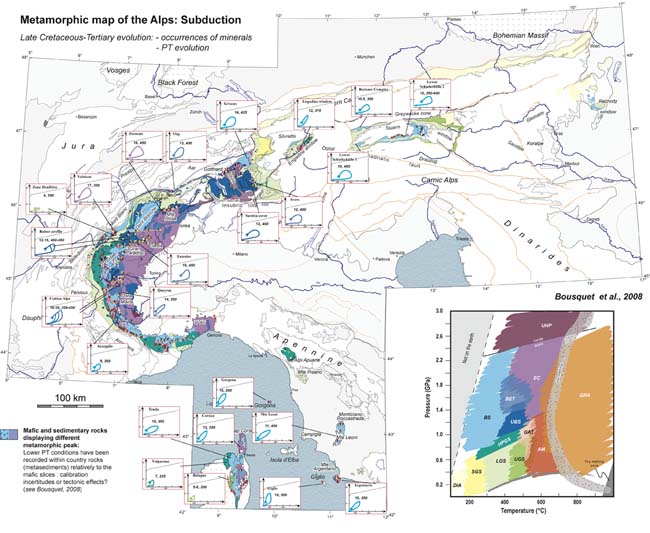 Figure 6: Mineral distribution (and some associated PT paths) of subduction-related processes in post-Hercynian metasediments of the Alps. We note a wide distribution of HP events over the entire alpine edifice. See references in Appendix |
We recognize the HP-LT imprint on the European continental margin (Tauern window, Adula nappe and surrounding covers), on all the metasediments derived from the partly oceanic Valaisan domain, as well as on the Piemont-Ligurian realm (Rechnitz window, Matrei zone, Avers, Zermatt-Saas zone, Entrelor, Cottian Alps, Voltri group, Tuscany and Corsica). The situation is more complex for the units derived from the Briançonnais terrane. They indicate, going from internal to external, eclogite and UHP conditions in the “internal massifs” (Monte Rosa, Gran Paradiso, Dora Maira), blueschist conditions (Suretta cover, Mont Fort, Ruitor, Vanoise, Acceglio, Ligurian Alps, Tenda), and subduction-related greenschist and low-grade conditions (Tasna, Schams, Zone Houillère). Based on structural arguments, some authors dispose of this complexity by changing the paleogeographic attribution of the “internal massifs” (Froitzheim 2001; Pleuger et al 2005). We are agreeing with the fact that the geodynamic evolution is complex and that the “internal massifs” are part of the Briançonnais (see for example Polino et al. 1990; Borghi et al. 1996; Froitzheim et al. 1996, Dal Piaz 1999).
In the following we will present four examples (Cottian Alps, Ruitor, Entrelor, Valais ocean) that document and demonstrate the use of metamorphic studies on metasediments for unraveling different PT evolutions during the early subduction-related history of the Alps.
Continuous P increase within sediments from one single paleogeographic unit (Cottian Alps)
The Schistes Lustrés complex in the Cottian Alps is formed by intensely folded Upper Jurassic (Malm; De Wever & Caby 1981) to Upper Cretaceous calcschists deposited in the oceanic Piemont-Liguria trough (Coniacian-Santonian; Lemoine and Tricard 1986, Deville et al. 1992), with a few mantle slivers (mainly serpentinites) representing the floor of this Alpine realm largely devoid of mafic oceanic crust (Lagabrielle & Lemoine 1997). The study of metamorphic sediments shows that carpholite-bearing assemblages are present in the western part (Goffé & Chopin, 1986; Agard et al., 2001) while chloritoid-bearing assemblages as well as garnet-lawsonite-galucophane assemblages in marbles (Ballèvre and Lagabrielle 1994), occur in the eastern part. On the basis of metapelite mineralogy, P-T estimates at maximum pressure increase from west to east across the study area from c. 1.2-1.3 GPa at 300-350°C (Agard et al. 2001) to 1.4-1.5 GPa at 450-500°C (Ballèvre and Lagabrielle 1994; Agard et al. 2000).
Bimodal evolution within a single paleogeographic unit (Ruitor area)
The metamorphic evolution of the basement units derived from the Briançonnais microcontinent was always a matter of debate (Desmons et al. 1999; Monié 1990). In the southwestern Alps, a HP imprint is well documented by occurrences of Fe,Mg-carpholite (Goffé 1977, 1984; Goffé et al. 1973, 2004; Goffé & Chopin 1986), and aragonite (Gillet & Goffé 1984) in metasediments and by occurrences of lawsonite and jadeite in metabasites (Lefèvre & Michard 1976; Schwartz et al. 2000). In the northwestern Alps in contrary, only the uppermost unit of the Briançonnais domain (the Mont Fort nappe) displays blueschist facies conditions (Schaer 1959, Bearth 1963).
In the Zone Houillère and in its Permo-Triassic cover as well as in the Briançonnais basement, metamorphic mineral assemblages are mainly composed of white micas with varying chemical composition, chloritoid and garnet. This same assemblage may occur within different lithologies (meta-arkose, meta-pelite, meta-sandstone). The increase in metamorphic grade from greenschist facies conditions in the Northwest (Zone Houillère) to the transition between blueschist and eclogite facies conditions in the Southeast (Internal Briançonnais) is well documented (Bucher & Bousquet, 2007). A major discontinuity in metamorphic grade, as documented by a pressure gap of ~0.7 kbar, is located at a tectonic contact within the Briançonnais terrane, namely that between the Zone Houillère and Ruitor unit (Caby et al. 1978, Bucher et al. 2003).
Rock associations displaying different metamorphic peak conditions (Entrelor area)
Two types of metamorphic rocks (blueschist and eclogites) have been described in this area, which is part of the Piemont-Liguria units of the Western Alps (Dal Piaz 1999). Recently, the rock assemblage in the Entrelor area has been interpreted as a metamorphic mix, consisting of eclogitic rocks that were embedded into a blueschist facies matrix consisting of metapelites and greenstones (Bousquet, 2007). The two kinds of HP metamorphic rocks reveal different peak metamorphic conditions (1.2 GPa at 450°C vs. 2.3 GPa at 550°C); it is their contemporaneous exhumation within a subduction channel which juxtaposed them at a shallower crustal level. This evolution illustrates that subduction processes cannot be considered as a single pass process; instead, return flow of a considerable portion of crustal and upper mantle material must be accounted for (Gerya et al. 2002), and the exhumation of the different rock types cannot be considered independently from each other (Engi et al. 2001). The rocks of the Entrelor area can be viewed as an exhumed part of a frozen subduction channel consisting of a mix of metamorphic rocks that have different metamorphic evolutions, and which were accreted at great depths.
Geometry of the subduction (units derived from the Valais ocean)
In the Eastern and Central Alps blueschist-facies rocks derived from the Valais ocean are exposed structurally below the Austroalpine nappes over an area of 300 x 20 km2 (from the Tauern window to the Grisons area) and have a thickness of around 10 km. This large volume of blueschist-facies rocks is in contrast with that of the eclogite-facies rocks of the Western Alps that only form a small 2 to 5 km thick slice. The difference in volume and metamorphic conditions from east to west is probably due to a change in style and geometry of subduction.
In the Eastern and Central Alps, the blueschist metasediments formed within a wide accretionary wedge with a thickness of 40-50 km which underlies the orogenic lid formed by the Austroalpine nappes and they were exhumed before the final collision between the European and Apulian continents (see discussion in Bousquet et al. 2002). Subduction occurred at a high angle to the strike of the orogen. In the Western Alps, where only a narrow accretionary wedge formed (Ceriani and Schmid, 2004), producing low-grade metamorphic conditions (Ceriani et al. 2003) subduction occurred in a sinistrally transpressive environment, i.e. at a small angle to strike of the orogen (Schmid & Kissling, 2000, Ceriani et al. 2001). The blueschist and eclogite facies metasediments of the Versoyen area (Petit St. Bernard and Versoyen units, Goffé and Bousquet 1997) were also subducted and extruded along a N-S direction, i.e. at a small angle to the orogen (Fügenschuh et al. 1999). Moreover, the Western Alps were never overlain by an orogenic lid formed by the Cretaceous-age Austroalpine nappe stack, but at best by rather thin basement slivers attributed to the Margna-Sesia fragment (Schmid et al. 2004).
Despite the fact that metamorphism related to Latest Cretaceous to Cenozoic subduction is scattered all over the Alps, information on these processes is unevenly distributed. Areas with wide occurrences of metasediments (Fig. 1) allow for the best insight the early geodynamic evolution of the Alps. In the Western Alps, all stages of a subduction process in PT frame (from UHP to greenschist conditions), as well its evolution in time (from the latest Cretaceous to the Oligocene, i.e. between 70 and 33 Ma) are recorded. Contrarily in the Eastern Alps and in the Tauern window (we exclude the Cretaceous-age high pressure metamorphism from this discussion since it was related to a different orogeny; Froitzheim et al., 1996) the metamorphic record of the meta-sediments is limited, and the HP rocks only exhibit Eocene ages (45-35 Ma).
Collision-related minerals apparently do not occur over the whole Alpine edifice. Minerals produced during collision are mainly indicative for temperature-dominated metamorphic conditions (Barrovian type metamorphism). They occur in the external zones, as well as in the Central Alps (Lepontine dome) and in the Tauern window of the Eastern Alps (Fig. 7).
click on the picture to zoom or click here to download the picture
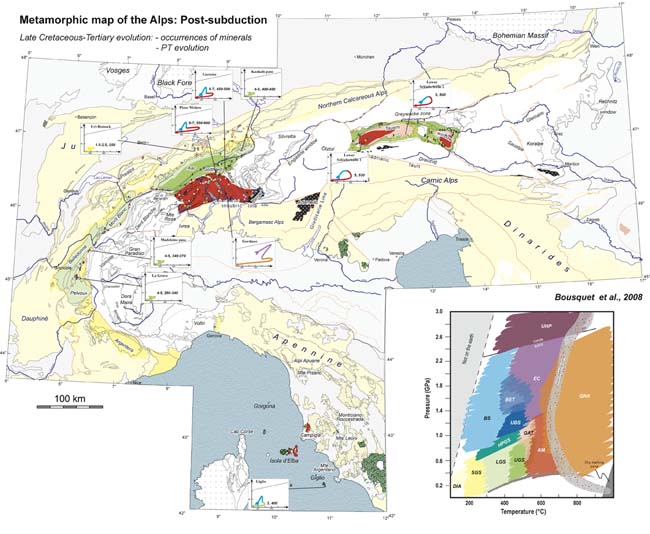 Figure 7: Mineral distribution (and some associated PT paths) of collision-related processes in post-Hercynian metasediments of the Alps. Two types of metamorphism can be distinguished. The lower one up to greenschist facies conditions has a large distribution all over the Alpine Belt and occurs in the external part. The second type is characterized by an important thermal event from greenschist to granulite conditions. It occurs only in three specific areas (Tauern, Lepontine dome and Tuscany). In these areas, the thermal event can overprint an earlier HP event. See references in Appendix. |
In the external zones, the metamorphic evolution reaches at maximum lower greenschist conditions, metamorphism resulting from collisional deformation as nappe emplacement, thrusting and folding (Frey, M. and Ferreiro-Mählmann 1999; Burkhard and Goy-Eggenberger 2001; Ferreiro-Mählmann, 2001). Burial processes both control metamorphic conditions in the external zones and limit it to low grade. The area of the Lepontine dome and the Tauern window, however, experienced higher metamorphic conditions, at least amphibolite facies.
Three examples will elucidate geodynamic processes that led to high temperature metamorphic overprinting of metasediments in more internal zones.
Continental underplating (Tauern window)
In the post-Variscan metasediments of the Tauern window a high temperature event is mainly indicated by the occurrence of Fe-Ca-rich garnet (Droop 1981; Selverstone 1985). Only a few occurrences of staurolite have been reported, indicating maximum amphibolite facies conditions. The mineral distribution pattern indicates two dome-like structures with concentric temperature gradients. This pattern resulted from the underthrusting of Europe-derived continental basement (Kurz et al. 1999) under and its accretion to the overlying Austroalpine basement complex (Apulian plate). This geodynamic scenario led to simple and continuous PT-paths (Fig. 6) that indicate decompressional heating from HP conditions into amphibolite facies conditions.
In Schierfehülle, the earlier HP-stage (blueschist facies conditions, Selverstone and Spear 1985) at 36 Ma was overprinted by HT conditions (amphibolite facies conditions) at around 30-27 Ma ago (Christensen et al. 1994; Zimmermann et al. 1994).
Continental wedging (Lepontine Dome)
High-temperature metamorphic conditions in the Central Alps (the Lepontine area) were based on the study of metasediments in the early works on Alpine geology already (e.g. Schmidt and Preiswerk 1908; Preiswerk 1918). Since these pioneering descriptions, several workers have dealt with meta-sediments from the Lepontine in order to understand progressive metamorphic evolution in isochemical systems (siliceous carbonates system: Trommsdorff 1966; pelitic and marly compositions: Frey 1969). The Lepontine area is characterized by extensive amphibolite facies conditions, reaching migmatization and/or granulite facies conditions. The thermal overprint (Fig. 6) progressively decreases from UHT-conditions in the south to greenschist facies conditions outwards (Streckeisen et al. 1974; Engi et al. 1995; Todd and Engi 1997). The northern margin of the amphibolite grade Lepontine dome is defined by the appearance of staurolite in pelitic systems. However, to the south it is truncated by the Insubric line, along which granulites and migmatites are juxtaposed to rocks of the Southern Alps that did not experience substantial Alpine metamorphism.
Thin Mesozoic metasedimentary bands separate large volumes of basement rocks belonging to various nappes stacked below the Austroalpine nappes and in front of the Southern Alps (Apulian plate). The accretion of vast amounts of crustal material derived from the European margin (Adula, Simano, Leventina) and the Briançonnais terrane (i.e. Maggia nappe) allowed for high radiogenic heat production (Verdoya et al. 2001; Roselle et al. 2002) producing HT assemblages.
The PT paths deduced from the high-grade metasediments often, but not always (see Nagel et al 2002.; Keller et al. 2005a for more simple PT paths) show bimodal trends (Fig. 6): a HP event is followed by a phase of heating (Engi et al. 2001; Berger et al. 2005; Brouwer and Engi, 2005; Brouwer et al. 2005; Wiederkehr et al. 2007). Shortly after the HP event, which probably occurred at around 45 Ma (47-51 Ma Bucher 2003; 42.6 Lapen et al. 2007 see discussion in Berger and Bousquet, this volume), HT metamorphic conditions prevailed over a long period of time until 30 Ma ago in the south, respectively 15 Ma in the north (Hunziker et al. 1992).
Post-orogenic heating (Tuscany)
In Western Tuscany, Quaternary magmatism is witnessed by volcanic as well as intrusive rocks. This magmatism is associated with crustal thinning and high heat flow values (Scrocca et al. 2003). Consequently, metasediments not only exhibit HP mineral assemblages, but also minerals such as andalusite, staurolite, chloritoide, epidote that document the high temperature evolution. A bimodal PT path has been reconstructed from Giglio, indicating both a HP and a HT event. (Rossetti et al. 1999).The inferred paleo-temperature distribution pattern resembles an asymmetric thermal high defined by the appearance of kyanite, similar to the present geothermal pattern of the Tuscan crust (Franchescelli et al. 1986), as indicated by a series of geothermal anomalies passing through the Northern Apennines (Della Vedoya et al. 2001). The age of the HT event (from 15 to 8 Ma, Kligfield et al. 1986; Brunet et al. 2000, Molli et al. 2000a, b) clearly post-dated the HP stage (31-26.7 Ma Brunet et al. 2000) in Tuscany.
Summary
Remnants of the high temperature event are unevenly distributed throughout the Alps. They are localized in the Tauern window, the Lepontine Dome, and in Tuscany. In contrast large areas lack such a HT overprint: the entire Western Alps and the Engadine window located between Lepontine dome and Tauern window. Both the Lepontine and the Tauern domes are made up of continent-derived granitoid upper crustal metamorphic sequences (Lammerer & Weger 1998; Neubauer et al. 1999; Schmid et al. 1996), while the Engadine window (Bousquet et al. 2002) and the Southwestern Alps (Agard et al. 2002; Lardeaux et al. 2006) are mainly built up of oceanic-metasedimentary sequences.Hence we conclude that the high temperature event in the Eastern and Central Alps is due to large local accumulations of crustal material during continental collision , while in Tuscany it records a post-orogenic event, associated to thinning of the lithosphere (Rossetti et al. 1999).
Sediments occur throughout mountain belts such as the Alps and represent a large variety of paleo-environments and chemical compositions. Moreover, several of these compositions are very sensitive to temperature and pressure variations. Thus they have a high potential for registering the different stages of their geodynamic evolution. Mineral distributions in metasediments combined with previous works on metabasites allow to decipher the complexity of the late Cretaceous-Tertiary alpine history (Fig. 8 and Fig. 9).
click on the picture to zoom or click here to download the picture
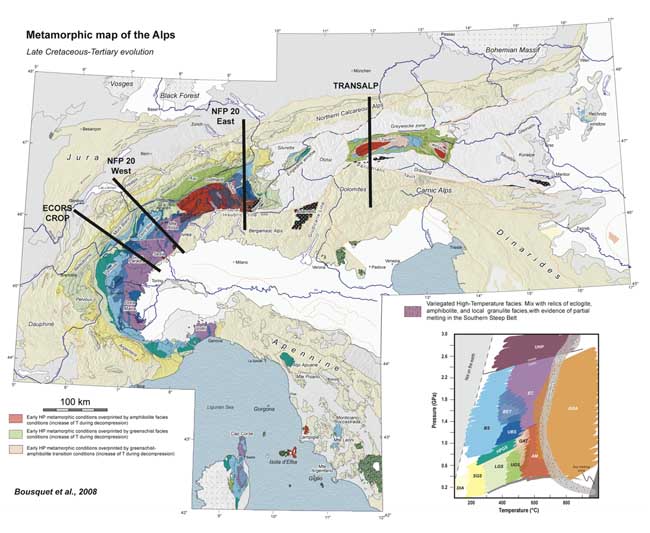 Figure 8: Metamorphic facies distribution (and related magmatism and volcanism) in the Alps from Vienna (Austria) to Corsica and Tuscany. On the map, the different stages of the geodynamic evolution are indicated. This map, largely modified from Oberhänsli et al. (2004) is a synthesis of figure 6 and 7. Traces of profiles presented on the figure 9 are indicated. |
Map representation (Fig 8) allows to clearly separated different areas in the Alps. Corsica, the Western Alps as well as the far eastern Alps (Rechnitz window) have recorded only the subduction-related evolution, characterized by HP metamorphism. The Central Alps and the Eastern Alps (Tauern window) are displaying a more complex history. In these areas the HP phase is overprinted by higher temperature conditions. Alpine eclogite facies remnants in the central Lepontine area appear to be restricted to a metamorphic mix (Berger et al 2007). They are isolated occurrences in a belt that includes relics of variegated high-grade metamorphism, from granulite facies to eclogite to amphibolite facies. This structure is interpreted as representing remnants of a tectonic accretion channel (Engi et al. 2001), which had developed along the convergent plate boundary during Alpine subduction.
From the metamorphic map (Fig 8) and using four major geological transects (Schmid et al 2004), we propose metamorphic transects across the Alps down to 15-20 km depth (Fig 9). In the eastern transect (Fig 9a, along the TRANSALP profile), the main alpine metamorphic features show the thermal overprint. Only scarce relics of the HP history are preserved. In the Central Alps (Fig 9b, NFP 20 east profile), HP and HT metamorphic rocks coexist. The thermal overprinting of different subduction patterns can be observed: eclogites of the Adula complex, rocks undergone into blueschist conditions (European margin -North of Adula, Simano-, Valais, Briançonnais -Tambo, Suretta-) as well as rocks that have not been subducted (Maggia nappe for the Briançonnais) have been thermally overprinted by the late Tertiary event. A wedge-type structure built against the Insubric Line can be clearly distinguished on the Central Alps profile. In the northwestern Alps (Fig 9c, NFP 20 west profile), while the subduction-related metamorphism is widespread, the thermal overprinting is limited to the European platform (sediments -Helvetic nappes or Dauphiné-, basement rocks - e.g. Mt Blanc-) or to the structurally lower units (root of the Mte Rosa, Antrona). In the Western Alps (Fig 9d, ECORS-CROP profile), subduction-related metamorphism is the main record. The thermal overprint appears west of the Penninic front and it is limited to the European platform (Belledonne, Pelvoux, Dauphiné). The most internal units are completely lacking a HT event. It can only be assumed for the deepest units (at around 20 km depth). Two subduction zones, indicated by HP metamorphic conditions, can be clearly evidenced. One (in the East) is formed by the Piemont-Liguria rocks, the Gran Paradiso massif and the most internal part of the Briançonnais. The second subduction zone is formed only by the Valais rocks and is rooted at depth. Both zones are separated from each other by the external Briançonnais (zone Houillère) which lacks evidence for HP metamorphism. The arrangement of the nappe pile in the Western Alps clearly shows a subduction-type structure in which most of the tectonic units dip southwestward.
click on the picture to zoom or click here to download the picture
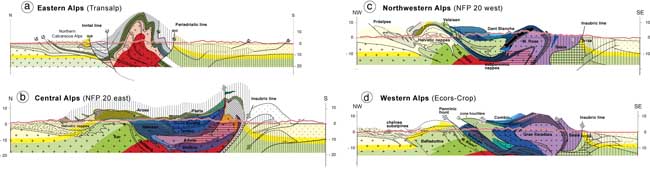 Figure 9: Metamorphic structure of the Alps. We report metamorphic data along four geological transects of Schmid et al (2004) down to 15-20 km depth. Below this depth, the past metamorphic history should be overprinted by the present thermal regime. a) Eastern transect along the TRANSALP seismic profile. b) Central Alps transect (along the NFP 20 east seismic profile . c) Northwestern Alps transect along the NFP 20 west seismic profile. d) Western Alps. transect, along the ECORS_CROP seismic profile. |
Evidence for HP metamorphism recording prolonged subduction processes during at least over 37 Ma (from 70 Ma in Sesia to 33 Ma in Valaisan, see Bousquet et al. 2002 and Berger and Bousquet, this volume for timing constraints). The evidence for these long lasting process is also widespread over the entire orogen, from the Rechnitz window in the East to Corsica and Tuscany in the Southwest. All paleogeographic units, of continental and oceanic origin, were involved in these subduction processes. The nappes derived from the Penninic-Austrolpine transition zone (Margna-Sesia fragment), the Piemont-Ligurian ocean, the Briançonnais terrane, the Valais ocean and the European margin all were successively subducted under the Apulian plate (Berger and Bousquet, this volume).
In contrast, high-temperature metamorphism a relative short-lived process, lasting for some 15 Ma (30 to 15 Ma). The evidence for such HT metamorphism are also localized in specific regions. It is limited to areas where considerable amounts of continental crust were accumulated into accreted nappe stacks. High temperature conditions (more than 650°C, up to 800°C; granulites and migmatites) were reached in the Lepontine dome where a huge amount of continental crust allowed for high radiogenic heat production. In the Tauern, a less important amount of imbricated continental crust led to amphibolite facies conditions (up to 600°C). In the Engadine window (Hitz 1995, 1996), as well as in the Western Alps, the relative scarcity of continental crust involved in the orogenic wedge does not allow for such a high heat production and associated high temperature overprint.
The transition between the Western Alps, lacking such a HT overprint, and the Lepontine dome can be observed in the Monte Rosa area. There, only the lower parts exposed in deep valleys (Domodossola) show an amphibolite facies overprint (650°C, Keller et al. 2005b).
Thermal overprint is primarily related to the amount of crust involved in the subduction and collision processes (Bousquet et al. 1997; Goffé et al. 2003) rather than to processes of shear or viscous heating (Burg and Gerya, 2005). The latter mechanism, which suppose high deformation rate will not allow for the preservation of HP-LT assemblages within high-grade rocks, as is found for example in the southern Adula complex (Nagel et al. 2002).
The relation between the volume of continental crust imbricated and intensity of high-temperature orogenic metamorphism can be generalized over the entire alpine edifice, except for Tuscany where the late (< 8 Ma) thermal overprint is clearly related to lithospheric thinning.
Conclusions
Based on metamorphic studies in metasediments, we evidence substantial differences in the metamorphic, and hence the geodynamical evolution along strike the Alpine orogen.
The Western Alps did not reach the mature stage of a head-on colliding belt as is indicated by a continuous metamorphic evolution, representing all the subduction related processes ranging from lower greenschist to UHP conditions. All the metamorphic rocks behind the Pennine frontal thrust were already exhumed to upper crustal level during ongoing oceanic and continental subduction and before collision with the Dauphinois domain from around 32 Ma onwards (Fügenschuh and Schmid, 2003; Leloup et al 2005). Hence, the Western Alps represent a frozen-in subduction zone. Since then only exhumation by erosional processes affected the inner parts of the orogen.
The rest of the Alpine orogen later underwent a more important collision process due to the ongoing head-on geometry of subduction and collision. It therefore often but not always shows a bimodal metamorphic evolution with two distinct P and T peaks. The intensity of the thermal overprint relates to the amount of crustal material incorporated to the orogenic wedge.
Acknowledgments
This article benefits of discussions with many colleagues. All cannot be cited, but RB wants to specially thank M. Ballèvre, B. Fügenschuh, S. Bucher, R. Caby, R. Polino, D. Marquer, G. Gosso, M.-I. Spalla, V. Höck, O. Vanderhaeghe, S. Duchene, R. Ferreiro-Mählmann, J.-M. Lardeaux and P. Rossi. We are indebted to N. Froitzheim for his corrections and comments. He helps us to clarify and improve the manuscript. Despite the arrogant tone of his review, we thank K. Stüwe. Some of his comments were useful. Without the encouragements, the editorial work, and the patience of S. Siegesmund this article would not be existed.
Appendix
List of the references used to build the maps on the figures 5, 6 and 7.
Eastern Alps: Bousquet (this study), Bousquet et al. (1998), Dachs (1986, 1990), Droop (1981, 1985), Franz (1983), Höck (1974, 1980), Leimser & Purtscheller (1980), Matile & Widmer (1993), Miller et al. (2007)
Central Alps: Bousquet (this study), Bousquet et al. (2002), Droop and Bucher-Nurminen (1984), Frey (1972, 1974), Frey and Wiedeland (1975), Frey et al. (1980), Frey & Ferreiro-Mählmann (1999), Goffé & Oberhänsli (1992), Irouschek (1983), Oberhänsli et al. (1995, 2003), Niggli & Niggli (1965), Staub (1926), Trommsdorff (1966), Wiederkehr et al. (2007)
Western Alps: Agard et al. (2000, 2001), Ballèvre (1988), Ballèvre and Lagabrielle (1994), Bousquet (2007), Bousquet et al. (2004), Bucher et Bousquet (2007), Caron & Saliot (1969), Caron (1974), Ceriani et al. (2003), Chopin (1981, 1984), Chopin et al. (2003), Cigolini (1995), Desmons et al. (1999), Gillet & Goffé (1988), Goffé (unpub.), Goffé et al. (1973), Goffé (1977, 1982, 1984), Goffé & Chopin (1986), Goffé & Velde (1984), Goffé & Bousquet (1997), Goffé at al. (2004), Henry (1990), Jullien & Goffé (1993), Le Bayon et al. (2006), Leikine et al. (1983), Martinotti (unpub.), Saliot (1979), Venturini (1995)Corsica and Tuscany: Caron & Péquignot (1986), Caron (1994), Daniel & Jolivet (1995), Daniel et al. (1996), Franceschelli et al. (1986, 1989), Franseschelli and Memmi (1999), Giorgetti et al. (1997), Goffé (unpub.), Goffé (1982), Jolivet et al. (1998), Leoni et al. (1996), Molli et al. (2006), Rossetti et al. (1999, 2001), Theye et al. (1997), Tribuzio and Giacomini (2002).
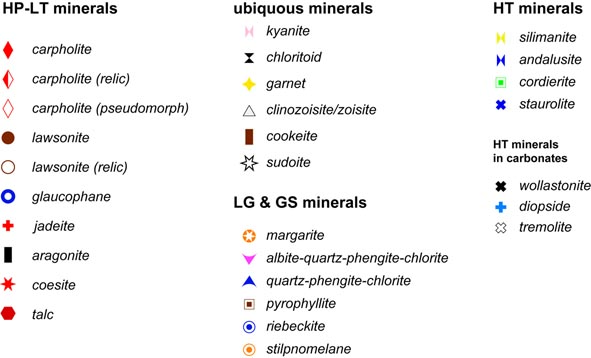 Figure appendix: list of the metamorphic minerals found in metasediments of the Alps (Table 2 in the original version of the paper) |
Agard, P., Goffé, B., Touret, J. L. R. & Vidal, O., 2000. Retrograde fluid evolution in blueschist-facies metapelites (Schistes lustrés unit, Western Alps). Contributions to Mineralogy and Petrology, 140, 296-315.Agard, P., Jolivet, L. & Goffé, B., 2001. Tectonometamorphic evolution of the Schistes Lustrés complex: implications for the exhumation of HP and UHP rocks in the Western Alps. Bulletin de la Société géologique de France, 172(5), 617-636.Agard, P., Monié, P., Jolivet, L. & Goffé, B., 2002. Exhumation of the Schistes Lustrés complex: in situ laser probe 40Ar/39Ar constraints and implications for the Western Alps. Journal of Metamorphic Geology, 20(6), 599-618.Ballèvre, M., 1988. Collision continentale et chemins P-T : l'unité pennique du Grand Paradis, Alpes Occidentales Centre Armoricain d'études structurale des socles, Rennes.Ballevre, M. & Lagabrielle, Y., 1994. Garnet in Blueschist-facies marbles from the Queyras unit (Western Alps) - its occurrence and its significance. Schweizerische Mineralogische Und Petrographische Mitteilungen, 74(2), 203-212.Barrow, G., 1893. On an intrusion of muscovitibiotite gneiss in the south-eastern Highlands of Scotland, and its accompanying metamorphism. The Quarterly Journal of the Geological Society, 49, 330-358.Barrow, G., 1912. On the geology of the lower Deeside and the souther Highland border. Proceedings of the Geologists' Association, 23, 268-284.Bearth, P., 1963. Contribution à la subdivision tectonique et stratigraphique du cristallin de la nappe du Grand Saint-Bernard dans le Valais (Suisse). In: Livre à la mémoire du Professeur Fallot (ed Durand Delga, M.), pp. 407-418, Mémoire de la Société géologique de France, Paris.Berger, A. & Bousquet, R., 2007. Subduction related metamorphism in the Alps: Review of isotopic ages based on petrology and their geodynamic consequences.Berger, A., Mercoli, I. & Engi, M., 2005. The central Lepontine Alps: Explanatory notes accompanying the tectonic-geological map sheet Sopra Ceneri (1:100′000). Schweizerische Mineralogische Und Petrographische Mitteilungen, 85(2/3), 109-146.Berman, R. G., 1988. Internally-Consistent Thermodynamic Data for Minerals in the system Na2O-K2O-Ca-MgO-FeO-Fe2O3-Al2O3-SiO2-TiO2-H2O-CO2. Journal of Petrology, 29(2), 445-522.Bigi, G., Castellarin, A., Coli, M., Dal Piaz, G. V., Sartori, R., Scandone, P. & Vai, G. B., 1990. Structural model of Italy. In: Progetto Geodinamica, SELCA, Firenze.Bousquet, R., 2007. Metamorphic heterogeneities within a same HP unit: overprint effect or metamorphic mix? Lithos, in press.Bousquet, R., Engi, M., Gosso, G., Oberhänsli, R., Berger, A., Spalla, M. I., Zucali, M. & Goffé, B., 2004. Transition from the Western to the Central Alps. In: Explanatory note to the map "Metamorphic structure of the Alps" (ed Oberhänsli, R.), pp. 145-156, Mitteilungen Österreichische Mineralogischen Gesellschaft, Wien.Bousquet, R., Goffé, B., Henry, P., Le Pichon, X. & Chopin, C., 1997. Kinematic, Thermal and Petrological model of the Central Alps: Lepontine Metamorphism in the Upper Crust and Eclogitisation of the Lower Crust. Tectonophysics, 273, 105-127.Bousquet, R., Goffé, B., Vidal, O., Oberhänsli, R. & Patriat, M., 2002. The tectono-metamorphic history of the Valaisan domain from the Western to the Central Alps: New constraints for the evolution of the Alps. Bulletin of the Geological Society of America 114(2), 207-225.Bousquet, R., Oberhänsli, R., Goffé, B., Jolivet, L. & Vidal, O., 1998. High pressure-low temperature metamorphism and deformation in the Bündnerschiefer of the Engadine window: implications for the regional evolution of the eastern Central Alps. Journal of Metamorphic Geology, 16, 657-674.Brouwer, F. M., Burri, T., Engi, M. & Berger, A., 2005. Eclogite relics in the Central Alps: PT-evolution, Lu-Hf ages, and implications for formation of tectonic mélange zones. Schweizerische Mineralogische Und Petrographische Mitteilungen, 85(2/3), 147-174.Bucher, K. & Frey, M., 1994. Petrogenesis of metamorphic rocks. Springer Verlag, Berlin.Bucher, S., 2003. The Briançonnais units along the ECORS-CROP transect (Italian-French Alps): structures, metamorphism and geochronology, Universität Basel, Basel.Bucher, S. & Bousquet, R., 2007. Metamorphic evolution of the Briançonnais units along the ECORS-CROP profile (Western Alps): New data on metasedimentary rocks. Journal of Swiss Geosciences, 100, (2), 1-16.Burg, J.-P. & Gerya, T. V., 2005. The role of viscous heating in Barrovian metamorphism of collisional orogens: thermomechanical models and application to the Lepontine Dome in the Central Alps. Journal of Metamorphic Geology, 23(2), 75-95.Caby, R., 1996. Low-angle extrusion of high-pressure rocks and the balance between outward and inward displacements of Middle Penninic units in the western Alps. Eclogae geologicae Helvetiae, 89(1), 229-267.Caron, J.-M., 1974. Rapports entre diverses 'générations' de lawsonite et les déformations dans les Schistes lustrés des Alpes cottiennes septentrionales (France et Italie). Bulletin de la Société Géologique de France, 16, 255-268.Caron, J.-M., 1994. Metamorphism and deformation in Alpine Corsica. Schweizerische Mineralogische Und Petrographische Mitteilungen, 74(1), 105-114.Caron, J.-M. & Pequignot, G., 1986. The Transition between Blueschists and Lawsonite-Bearing Eclogites Based on Observations from Corsican Metabasalts. Lithos, 19(3-4), 205-218.Caron, J.-M. & Saliot, P., 1969. Nouveaux gisements de lawsonite et de jadeite dans les alpes franco-italiennes. Comptes Rendus de l'Académie des Sciences Paris, 268, 3153-3156.Carswell, D. A., 1990. Eclogite Facies Rocks. Blackie Academic and Professional, London.Ceriani, S., Fugenschuh, B., Potel, S. & Schmid, S. M., 2003. Tectono-metamorphic evolution of the Frontal Penninic units of the Western Alps: correlation between low-grade metamorphism and tectonic phases. Schweizerische Mineralogische Und Petrographische Mitteilungen, 83(2), 111-131.Ceriani, S., Fügenschuh, B. & Schmid, S. M., 2001. Multi-stage thrusting at the "Penninic Front" in the Western Alps between Mont Blanc and Pelvoux massifs. International Journal of Earth Sciences, 90(3), 685-702.Ceriani, S. & Schmid, S. M., 2004. From N-S collision to WNW-directed post-collisional thrusting and folding: Structural study of the Frontal Penninic Units in Savoie (Western Alps, France) Eclogae Geologicae Helvetiae, 97, 347-369.Chatterjee, N. D., 1976. Margarite stability and compatibility relations in the system CaO-Al2O3-SiO2-H2O as a pressure-temperature indicator. American Mineralogist, 61, 699-709.Chopin, C., 1981. Talc-phengite: a Widespread Assemblage in High-Grade Pelitic Blueschists of the Western Alps. Journal of Petrology, 22(4), 628-650.Chopin, C., 1984. Coesite and pure pyrope in high grade blueschists of the western alps: a first records and some consequences. Contributions to Mineralogy and Petrology, 86, 107-118.Chopin, C., 1987. Very-High-Pressure Metamorphism in the Western Alps: Implications for Subduction of Continental Crust. Philosophical Transactions of the Royal Society of London, 321(1557), 183-195.Chopin, C., Goffé, B., Ungaretti, L. & Oberti, R., 2003. Magnesiostaurolite and zincosttaurolite: mineral description with a petrogenetic and crystal-chemical update. European Journal of Mineralogy, 15, 167-176.Chopin, C. & Schreyer, W., 1983. Magnesiocarpholite and magnesiochloritoid: two index minerals of pelitic blueschits and their preliminary phase relations in tne system MgO-Al2O3-SiO2-H2O. American Journal of Science, 283-A, 72-96.Chopin, C. & Sobolev, N. V., 1995. Principal mineralogic indicators of UHP in crustal rocks. In: Ultrahigh Pressure Metamorphism (eds Coleman, R. G. & Wang, X.), pp. 96-131, Cambridge University Press, Cambridge.Cigolini, C., 1995. Geology of the Internal Zone of the Grand Saint Bernard Nappe: a metamorphic Late Paleozoic volcano-sedimentary sequence in South-Western Aosta Valley (Western Alps). In: Studies on metamorphic rocks and minerals of the western Alps. A Volume in Memory of Ugo Pognante. (ed Lombardo, B.), pp. 293-328, Bollettino del Museo Regionale di Scienze Naturali (suppl.), Torino.Coleman, R. G. & Wang, X., 1995. Ultrahigh Pressure Metamorphism. Cambridge University Press, Cambridge.Dachs, E., 1986. High-pressure mineral assemblages and their breakdown-products in metasediments South of the Grossvenidiger, Tauern Window, Austria. Schweizerische Mineralogische Und Petrographische Mitteilungen, 66(1/2), 145-162.Dachs, E., 1990. Geothermobarometry in metasediments of the southern Grossvenediger area (Tauern Window, Austria). Journal of Metamorphic Geology, 8(2), 217-230.Dal Piaz, G. V., 1974a. Le métamorphisme alpin de haute pression-basse température dans l'évolution structurale du bassin ophiolitique alpino-appenninique. 1ère partie. Bolletino della Società Geologica Italiana, 93, 437-468.Dal Piaz, G. V., 1974b. Le métamorphisme alpin de haute pression-basse température dans l'évolution structurale du bassin ophiolitique alpino-appenninique. 2ème partie. Schweizerische Mineralogische und Petrographische Mitteilungen, 54, 399-424.Dal Piaz, G. V., 1999. The Austroalpine-Piedmont nappe stack and the puzzle of Alpine Tethys. In: 3rd Workshop on Alpine Geological Studies (eds Gosso, G., Jadoul, F., Sella, M. & Spalla, M. I.), pp. 155-176, Memorie di Scienze Geologiche, Padova.Dal Piaz, G. V., 2001. History of tectonic interpretations of the Alps. Journal of Geodynamics, 32, 99-114.Dal Piaz, G. V., Hunziker, J. C. & Martinotti, G., 1972. La Zona Sesia - Lanzo e l'evoluzione tettonico-metamorfica delle Alpi Nordoccidentali interne. Memorie della Societa Geologica Italiana, 11, 433-460.Daniel, J.-M. & Jolivet, L., 1995. Detachment faults and pluton emplacement - Elba Island (Tyrrhenian sea). Bulletin de la Société Géologique de France, 166(4), 341-354.Daniel, J. M., Jolivet, L., Goffé, B. & Poinssot, C., 1996. Crustal-scal strain partitionning: footwall deformation below the Alpine Oligo-Miocene detachment of Corsica. Journal of Structural Geology, 18(1), 41-59.De Capitani, C., 1994. Gleichgewichts-Phasendiagramme: Theorie und Software. Berichte der Deutschen Mineralogischen Gesellschaft, 6, 48.De Capitani, C. & Brown, T. H., 1987. The computation of chemical equilibrium in complex systems containing non-ideal solutions. Geochimica et Cosmochimica Acta, 51, 2639-2652.de Roever, W. P., 1951. Ferrocarpholite, the hitherto unknown ferrous iron analogue of capholite proper. American Mineralogist, 36, 736-745.De Wever, P. & Caby, R., 1981. Datation de la base des schistes lustrés postophiolitiques par des radiolaires (Oxfordien-Kimmeridgien moyen) dans les Alpes Cottiennes (Saint Véran, France). Comptes Rendus de L'Académie des Sciences, 292, 467-472.Della Vedoya, B., Bellani, S., G, P. & Squarci, P., 2001. Deep temperatures and surface heat flow distribution. In: natomy of an orogen: the Apenninesand adjacent Mediterranean basins (eds Vai, G. B. & Martini, P.), pp. 65-76, Kluwer Academic Publishers, Dordrechtt, The Netherlands.Desmons, J., Aprahamian, J., Compagnoni, R., Cortesogno, L. & Frey, M., 1999. Alpine Metamorphism of the Western Alps: I. Middle to highT/P metamorphism. Schweizerische Mineralogische und Petrographische Mitteilungen, 79, 89-110.Deville, E., Fudral, S., Lagabrielle, Y., Marthaler, M. & Sartori, M., 1992. From oceanic closure to continental collision: A synthesis of the "Schistes lustrés" metamorphic complex of the Western Alps. Bulletin of the Geological Society of America, 104(2), 127-139.Droop, G. T. R., 1981. Alpine metamorphism of pelitic schists in the Southeast Tauern Window, Austria. Schweizerische Mineralogische Und Petrographische Mitteilungen, 61, 237-273.Droop, G. T. R., 1985. Alpine metamorphism in the south-east Tauern Window, Austria: 1. P-T variations in space and time. Journal of Metamorphic Geology, 3, 371-402.Droop, G. T. R. & Bucher-Nurminen, K., 1984. Reaction Textures and Metamorphic Evolution of Sapphirine-bearing from Gruf Complex, Italian Central Alps. Journal of Petrology, 25(3), 766-803.Engi, M., Berger, A. & Roselle, G. T., 2001. Role of the accretion channel in collisional orogeny. Geology, 29(12), 1143-1146.Engi, M., Todd, C. S. & Schmatz, D. R., 1995. Tertiary metamorphic conditions in the eastern Lepontine Alps. Schweizerische Mineralogische und Petrographische Mitteilungen, 75, 347-369.Ernst, W. G., 1971. Metamorphic zonations on Presumably Subducted Lithospheric Plates from Japan, California and the Alps. Contributions to Mineralogy and Petrology, 34, 43-59.Escher, A. & Beaumont, C., 1997. Formation, burial and exhumation of basement nappes at crustal scale: a geometric model based on the Western Swiss-Italian Alps. Journal of Structural Geology, 19(7), 955-974.Eskola, P., 1929. Om mineral facies. Geologiska Föreningens i Stockholm Förhandlingar, 51(2), 157-172.Evans, B. W., 1990. Phase relation of epidote blueschists. Lithos, 25, 3-23.Franceschelli, M., Leoni, L., Memmi, I. & Puxeddu, M., 1986. Regional distribution of Al-silicates and metamorphic zonation in the low-grade Verrucano metasediments from the Northern Apennines, Italy. Journal of Metamorphic Geology, 4(3), 309-321.Franceschelli, M., Mellini, M., Memmi, I. & Ricci, C. A., 1989. Sudoite, a rock-forming mineral in Verrucano of the Northern Apennines (Italy) and the sudoite-chloritoid-pyrophyllite assemblage in prograde metamorphism. Contributions to Mineralogy and Petrology, 101(3), 274-279.Franceschelli, M. & Memmi, I., 1999. Zoning of chloritoid from kyanite-facies metapsammites, Alpi Apuane, Italy. Mineralogical Magazine, 63(1), 105-110.Franz, G. & Spear, F. S., 1983. High pressure metamorphism of siliceous dolomites from the central Tauern Window, Austria. American Journal of Science, 283-A, 396-413.Frey, M., 1969. Die Metamorphose des Keupers vom Tafeljura bis zum Lukmanier-Gebiet, Bern.Frey, M., 1978. Progressive Low-Grade Metamorphism of a Black Shale Formation, Central Swiss Alps, with Special Reference to Pyrophyllite and Margarite Bearing Assemblages. Journal of Petrology, 19(1), 95-135.Frey, M., 1978. Progressive low-grade metamorphism of a black shale formation, Central Swiss Alps, with special reference to pyrophyllite and margarite bearing assemblages. Journal of Petrology, 19(1), 95-135.Frey, M., Bucher, K., Frank, E. & Mullis, J., 1980. Alpine metamorphism along the GeoTraverse Basel-Chiasso -a review. Eclogae Geologicae Helvetiae, 73(2), 527-546.Frey, M., De Capitani, C. & Liou, J. G., 1991. A new petrogenetic grid for low-grade metabasites. Journal of Metamorphic Geology, 9, 497-509.Frey, M. & Ferreiro-Mählmann, R., 1999. Alpine metamorphism of the Central Alps. Schweizerische Mineralogische und Petrographische Mitteilungen, 79, 135-154.Frey, M., Hunziker, J.-C., Frank, W., Boquet, J., Dal Piaz, G. V., Jäger, E. & Nigli, E., 1974. Alpine metamorphic of the Alps a review. Schweizerische Mineralogische und Petrographische Mitteilungen, 54, 247-291.Frey, M., Hunziker, J. C., Roggwiller, P. & Schindler, C., 1973. Progressive niedriggradige Metamorphose glaukonitführender Horizonte in den helvetischen Alpen der Ostschweiz. Contributions to Mineralogy and Petrology, 39(3), 185-218.Frey, M. & Niggli, E., 1972. Margarite, an important mineral rock.forming mineral in regionally metamorphosed low-grade rocks. Naturwissenschaften, 59(5), 214-225.Frey, M. & Wieland, B., 1975. Chloritoid in autochthon-paraautochthonen Sedimenten des Aarmassivs. Schweizerische Mineralogische und Petrographische Mitteilungen, 55, 407-418.Fügenschuh, B., Loprieno, A., Ceriani, S. & Schmid, S. M., 1999. Structural analysis of the Subbriançonnais and Valais units in the area of Moùtiers (Savoy, Western Alps): paleogeographical and tectonic consequences. International Journal of Earth Sciences, 88, 201-218.Fügenschuh, B. & Schmid, S. M., 2003. Late stages of deformation and exhumation of an orogen constrained by fission-track: A case study in the Western Alps. Bulletin of the Geological Society of America, 115(11), 1425-1440.Gerya, T. V. & Stockhert, B., 2002. Exhumation rates of high pressure metamorphic rocks in subduction channels: The effect of Rheology. Geophysical Research Letters, 29(8), 1261.Gillet, P. & Goffé, B., 1988. On the signifiance of aragonite occurrence in the Western Alps. Contributions to Mineralogy and Petrology, 99, 70-81.Giorgetti, G., Memmi, I. & Nieto, F., 1997. Microstructures of intergrown phyllosilicate grains from Verrucano metasediments (northern Apennines, Italy). Contributions to Mineralogy and Petrology, 128(2-3), 127-138.Goffé, B., 1977. Succession de subfacies métamorphiques en Vanoise méridionale (Savoie). Contributions to Mineralogy and Petrology, 62, 23-41.Goffé, B., 1982. Définition du faciès à Fe,Mg-carpholite-chloritoide, un marqueur du métamorphisme de HP-BT dans les métasédiments alumineux, Paris, Université P. et M. Curie.Goffé, B., 1984. Le facies à carpholite-chloritoide dans la couverture briançonnaise des Alpes Ligures: un témoin de l'histoire tectono-metamorphique régionale. Memorie della Societa Geologica Italiana, 28, 461-479.Goffé, B. & Bousquet, R., 1997. Ferrocarpholite, chloritoïde et lawsonite dans les métapelites des unités du Versoyen et du Petit St Bernard (zone valaisanne, Alpes occidentales). Schweizerische Mineralogische und Petrographische Mitteilungen, 77, 137-147.Goffé, B., Bousquet, R., Henry, P. & Le Pichon, X., 2003. Effect of the chemical composition of the crust on the metamorphic evolution of orogenic wedges. Journal of Metamorphic Geology, 21(2), 123-142.Goffé, B. & Chopin, C., 1986. High-pressure metamorphism in the Western Alps: zoneography of metapelites, chronology and consequences. Schweizerische Mineralogische und Petrographische Mitteilungen, 66, 41-52.Goffé, B., Goffé-Urbano, G. & Saliot, P., 1973. Sur la présence d'une variété magnésienne de la ferrocarpholite en Vanoise (Alpes françaises) : sa signification probable dans le métamorphisme alpin. Comptes Rendus de l'Académie des Sciences Paris, 277, 1965-1968.Goffé, B. & Oberhänsli, R., 1992. Ferro- and magnesiocarpholite in the "Bündnerschiefer" of the eastern Central Alps (Grisons and Engadine Window). European Journal of Mineralogy, 4, 835-838.Goffé, B. & Velde, B., 1984. Contrasted metamorphic evolution in thrusted cover units of the Briançonnais zone (french Alps): a model for the conservation of HP-BT metamorphic mineral assemblages. Earth and Planetary Science Letters, 68, 351-360.Handy, M. & Oberhänsli, R., 2004. TAge Map of the Metamorphic Structure of the Alps - Tectonic Interpretation and Outstanding Problems. In: Explanatory note to the map "Metamorphic structure of the Alps" (ed Oberhänsli, R.), pp. 201-226, Mitteilungen Österreichische Mineralogischen Gesellschaft, Wien.Hauÿ, R.-J., 1822. Traité de minéralogie. Seconde édition, revue, corrigée et considérablement augmentée par l′auteur. Bachelier et Huzard, Paris.Hébert, R. & Ballèvre, M., 1993. Petrology of staurotide-bearing metapelites from the Cadomian belt, nothern Brittany (France): constraints on low-pressure metamorphism. Bulletin de la Société géologique de France, 164(2), 215-228.Henry, C., 1990. L′unité à coesite du massif de Dora maira dans son cadre pétrologique et structural (Alpes occidentals, Italie), Université Paris VI, Paris.Henry, P., Le Pichon, X. & Goffé, B., 1997. Kinematic, thermal and petrological model of the Himalayas: constraints related to metamorphism whithin the underthrust Indian crust and topographic elevation. Tectonophysics, 273, 31-56.Hitz, L., 1995. The 3D crustal structure of the Alps of eastern Switzerland and western Austria interpreted from a network of deep-seismic profiles. Tectonophysics 248, 71-96. Tectonophysics, 248, 71-96.Hitz, L., 1996. The deep structure of the Engadine Window: Evidence from deep seismic data. Eclogae Geologicae Helvetiae, 89(2), 657-675.Höck, V., 1974. Coexiting phengite,paragonite and margarite in metasediments of mittlere Hohe-Tauern, Austria. Contributions to Mineralogy and Petrology, 43(4), 261-273.Höck, V., 1980. Distribution maps of minerals of the Alpine metamorphism in the Penninic Tauern window, Austria. Mitteilungen österreichische geologische Gesellschaft, 71/72, 119-127.Hunziker, J. C., 1974. Rb-Sr and K-Ar age determination and the Alpine history of the Western Alps. Mem. Ist. Geol. Mineral. Univ. Padova, 31, 1-54.Irouschek, A., 1983. Mineralogie und Petrographie von Metapeliten der Simano-Decke unter besonderer Berücksichtigung cordieritführender Gesteine zwischen ALpe Sponda and Biasca, Universität Basel, Basel.Jullien, M. & Goffé, B., 1993. Occurrences de cookeite et de pyrophyllite dans les schistes du Dauphinois (Isère, France): Conséquences sur la répartition du métamorphisme dans les zones externes alpines. Schweizerische Mineralogische und Petrographische Mitteilungen, 73, 357-363.Keller, L. M., Hess, M., Fügenschuh, B. & Schmid, S. M., 2005a. Structural and metamorphic evolution of the Camughera- Moncucco, Antrona and Monte Rosa units southwest of the Simplon line, Western Alps. Eclogae Geologicae Helvetiae, 98, 19-49.Keller, L. M., Abart, R., Schmid, S. M. & De Capitani, C., 2005b. Phase Relations and Chemical Composition of Phengite and Paragonite in Pelitic Schists During Decompression: a Case Study from the Monte Rosa Nappe and Camughera-Moncucco Unit, Western Alps. Journal of Petrology, 46, 2145-2166.Kligfield, R., Hunziker, J., Dallmeyer, R. D. & Schamel, S., 1986. Dating of deformation phases unsing K-Ar and 40Ar/39Ar techniques - Results from the Northern Apennines. Journal of Structural Geology, 8(7), 781-798.Kohn, M. J. & Spear, F. S., 1993. Phase Equilibria of Margarite-Bearing Schists and Chloritoid + Hornblende Rocks form Western New Hampshire, USA. Journal of Petrology, 34(4), 631-651.Koons, P. O. & Thompson, A. B., 1985. No-mafic rocks in the greenschist, blueschist and eclogite facies. Chemical Geology, 50, 3-30.Kurz, W., Neubauer, F. & Unzog, W., 1999. Evolution of Alpine Eclogites in the Eastern Alps: Implications for Alpine Geodynamics. Physics and Chemistry of the Earth, part A: Solid Earth And Geodesy, 24(8), 667-674.Lammerer, B. & Weger, M., 1998. Footwall uplift in an orogenic wedge: the Tauern Window in the Eastern Alps of Europe. Tectonophysics, 285, 213-230.Lardeaux, J. M., Schwartz, S., Tricart, P., Paul, A., Guillot, S., Bethoux, N. & Masson, F., 2006. A crustal-scale cross-section of the south-western Alps combining geophysical and geological imagery. Terra Nova, 18(6), 412-422.Le Bayon, B., Pitra, P., Ballevre, M. & Bohn, M., 2006. Reconstructing P-T paths during continental collision using multi-stage garnet (Gran Paradiso nappe, Western Alps). Journal of Metamorphic Geology, 24(6), 477-496.Lefèvre, R. & Michard, A., 1965. La jadéite dans le métamorphisme alpin, à propos des gisements de type nouveau, de la bande d'Acceglio (Alpes cottiennes, Italie). Bulletin de la Société française de Minéralogie et de Cristallographie, LXXXVIII, 664-677.Leikine, M., Kienast, J.-R., Eltchaninoff-Lancelot, C. & Triboulet, S., 1983. Le métamorphisme polyphasé des unités dauphinoises entre Belledonne et Mont-Blanc (Alpes occidentales). Relation avec les épisodes de déformation. Bulletin de la Société géologique de France, XXV, 575-587.Leimser, W. M. & Purtscheller, F., 1980. Beiträge zur Metamorphose von Metvulkaniten in Pennin des Engadiner Fenster. Mitteilungen österreichische geologische Geselschaft, 71/72, 129-137.Leloup, P. H., Arnaud, N., Sobel, E. R. & Lacassin, R., 2005. Alpine thermal and structural evolution of the highest external crystalline massif: The Mont Blanc. Tectonics, 24(4), TC4002.Lemoine, M. & Tricart, P., 1986. Les schistes lustrés piémontais des Alpes Occidentales: Approches stratigraphique, structurale et sédimentologique. Eclogae geologicae Helvetiae, 79(2), 271-294.Leoni, L., Marroni, M., Sartori, F. & Tamponi, M., 1996. Metamorphic grade in the metapelites of the Internal Liguride Units (Northern Apennines, Italy). European Journal of Mineralogy, 8, 35-50.Massonne, H.-J. & Schreyer, W., 1987. Phengite geobarometry based on the limiting assemblage with K-feldspar, phlogopite and quartz. Contributions to Mineralogy and Petrology, 96, 212-224.Massonne, H.-J. & Szpurka, Z., 1997. Thermodynamic properties of white micas on the basis of high-pressure experiments in the systems K2O-MgO-Al2O3-SiO2-H2O and K2O-FeO-Al2O3-SiO2-H2O. Lithos, 41, 229-250.Massonne, H. J. & O'Brien, P. J., 2003. The Bohemian Massif and the NW Himalaya. In: Ultrahigh Pressure Metamorphism (eds Carson, C. J. & Compagnoni, R.), pp. 145-187, Eötvös University Press, Budapest.Matile, L. & Widmer, T. W., 1993. Contact-metamorphism of siliceous dolomites marls and pelites in the SE contact aureole of the Bruffione intrusion (SE Adamello, N Italy). Schweizerische Mineralogische Und Petrographische Mitteilungen, 73(1), 53-67.McDade, P. & Harley, S. L., 2001. A petrogenetic grid for aluminous granulite facies metapelites in the KFMASH system. Journal of Metamorphic Geology, 19(1), 45-60.Miller, C., Konzett, J., Tiepolo, M., Armstrong, R. A. & Thöni, M., 2007. Jadeite-gneiss from the Eclogite Zone, Tauern Window, Eastern Alps, Austria: Metamorphic, geochemical and zircon record of a sedimentary protolith. Lithos, 93(1-2), 68-88.Molli, G., Conti, P., Giorgetti, G., Meccheri, M. & Oesterling, N., 2000a. Microfabric study on the deformational and thermal history of the Alpi Apuane marbles (Carrara marbles), Italy. Journal of Structural Geology, 22, 1809-1825.Molli, G., Giorgetti, G. & Meccheri, M., 2000b. Structural and petrological constraints on the tectono- metamorphic evolution of the Massa Unit (Alpi Apuane, NW Tuscany, Italy). Geological Journal, 35(3-4), 251-264.Molli, G., Tribuzio, R. & Marquer, D., 2006. Deformation and metamorphism at the eastern border of the Tenda Massif (NE Corsica): a record of subduction and exhumation of continental crust. Journal of Structural Geology, 28(10), 1748-1766.Monié, P., 1990. Preservation of Hercynian Ar-40/Ar-39 Ages Through High-Pressure Low-Temperature Alpine Metamorphism In The We ustern Alps. European Journal of Mineralogy, 2(3), 343-361.Nagel, T., De Capitani, C., Frey, M., Froitzheim, N., Stünitz, H. & Schmid, S. M., 2002. Structural and metamorphic evolution in the Lepontine dome. Eclogae Geologicae Helvetiae, 95(3), 301-322.Neubauer, F., Genser, J., Kurz, W. & Wang, X., 1999. Exhumation of the Tauern window, Eastern Alps. Physics and Chemistry of the Earth, part A: Solid Earth And Geodesy, 24(8), 675-680.Niggli, E., 1970. Alpine Metamorphose und alpine Gebirgsbildung. Fortschritte der Mineralogie, 47, 16-26.Niggli, E. & Niggli, C., 1965. Karten der Verbreitung einiger Mineralien der alpidischen Metamorphose in den Schweizer Alpen (Stilpnomelan, Alkali-Amphibol, Chloritoid, Staurolith, Disthen, Sillimanit). Eclogae Geologicae Helvetiae, 58, 335-368.Oberhänsli, R., Bousquet, R., Engi, M., Goffé, B., Gosso, G., Handy, M., Höck, V., Koller, F., Lardeaux, J.-M., Polino, R., Rossi, P., Schuster, R., Schwartz, S. & Spalla, M. I., 2004. Metamorphic structure of the Alps. In: Explanatory note to the map "Metamorphic structure of the Alps", Commission for the Geological Map of the World, Paris.Oberhänsli, R., Bousquet, R. & Goffé, B., 2003. Comment to ”Chloritoid composition and formation in the eastern Central Alps: a comparison between Penninic and Helvetic occurrences“. Schweizerische Mineralogische und Petrographische Mitteilungen, in press.Oberhänsli, R., Goffé, B. & Bousquet, R., 1995. Record of a HP-LT metamorphic evolution in the Valais zone: Geodynamic implications. In: Studies on metamorphic rocks and minerals of the western Alps. A Volume in Memory of Ugo Pognante. (ed Lombardo, B.), pp. 221-239, Bollettino del Museo Regionale di Scienze Naturali (suppl.), Torino.Parra, T., Vidal, O. & Agard, P., 2002. A thermodynamic model for Fe-Mg dioctahedral K white micas using data from phase-equilibrium experiments and natural pelitic assemblages. Contributions to Mineralogy and Petrology, 143, 706-732.Parra, T., Vidal, O. & Jolivet, L., 2002. Relation between the intensity of deformation and retrogression in blueschist metapelites of Tinos Island (Greece) evidenced by chlorite-mica local equilibria. Lithos, 63(1-2), 41-66.Pattison, D. R. M., Spear, F. S., Debuhr, C. L., Cheney, J. T. & Guidotti, C. V., 2002. Thermodynamic modelling of the reaction muscovite+cordierite Al2SiO5+biotite+quartz+ H2O: constraints from natural assemblages and implications for the metapelitic petrogenetic grid. Journal of Metamorphic Geology, 20(1), 99-118.Pfiffner, O. A., Ellis, S. & Beaumont, C., 2000. Collision tectonics in the Swiss Alps: Insight from geodynamic modeling. Tectonics, 19(6), 1065-1094.Preiswerk, H., 1918. Geologische Beschreibung der Lepontinischen Alpen, Bern.Proyer, A., 2003. Metamorphism of pelites in NKFMASH a new petrogenetic grid with implications for the preservation of high-pressure mineral assemblages during exhumation. Journal of Metamorphic Geology, 21(5), 493-509.Rimmelé, G., Oberhänsli, R., Goffé, B., Jolivet, L., Candan, O. & Cetinkaplan, M., 2003. First evidence of high-pressure metamorphism in the "Cover Series" of the southern Menderes Massif. Tectonic and metamorphic implications for the evolution of SW Turkey. Lithos, 71(1), 19-46.Rimmele, G., Parra, T., Goffe, B., Oberhansli, R., Jolivet, L. & Candan, O., 2005. Exhumation Paths of High-Pressure-Low-Temperature Metamorphic Rocks from the Lycian Nappes and the Menderes Massif (SW Turkey): a Multi-Equilibrium Approach. Journal of Petrology, 46(3), 641-669.Roselle, G. T. & Engi, M., 2002. Ultra high pressure (UHP) terrains: Lessons from thermal modeling. American Journal of Science, 312(5), 410-441.Rossetti, F., Faccenna, C., Jolivet, L., Funiciello, R., Goffé, B., Tecce, F., Brunet, C., Monié, P. & Vidal, O., 2001. Structural signature and exhumation P-T-t path of the Gorgona blueschist sequence (Tuscan archipelago, Italy). Ofioliti, 26(2A), 175-186.Rossetti, F., Faccenna, C., Jolivet, L., Funiciello, R., Tecce, F. & Brunet, C., 1999. Syn- versus post-orogenic extension: the case study of Giglio Island (Northern Tyrrhenian Sea, Italy). Tectonophysics, 304, 71-93.Roure, F., Heitzmann, P. & Polino, R., 1990. Deep structure of the Alps. Mémoires de la société géologique de France, Paris.Saliot, P., 1979. La jadéite dans les Alpes françaises. Bulletin de la Société française de Minéralogie et de Cristallographie, 102, 391-401.Schaer, J.-P., 1959. Géologie de la partie septentrionale de l'éventail de Bagnes (entre le Val d'Hérémence et le Vaal de Bagnes, Valais, Suisse). Archives des Sciences (Genève), 12, 473-620.Schmid, S. M., Fugenschuh, B., Kissling, E. & Schuster, R., 2004. Tectonic map and overall architecture of the Alpine orogen. Eclogae Geologicae Helvetiae, 97(1), 93-117.Schmid, S. M. & Kissling, E., 2000. The arc of the Western Alps in the light of new data on deep crustal structure. Tectonics, 19(1), 62-85.Schmid, S. M., Pfiffner, O. A., Froitzheim, N., Schönborn, G. & Kissling, E., 1996. Geophysical-geological transect and tectonic evolution of the Swiss-Italian Alps. Tectonics, 15(5), 1036-1064.Schmidt, C. & Preiswerk, H., 1908. Geologische Karte der Simplongruppe. Spezialkarte n°48 mit Erläuterungen.Schwartz, S., Lardeaux, J.-M. & Tricart, P., 2000. La zone d'Acceglio (Alpes cottiennes): un nouvel exemple de croûte continentale éclogitisée dans les Alpes occidentales. Comptes Rendus de l'Académie des Sciences Paris, 320, 859-866.Scrocca, D., Doglioni, C. & Innocenti, F., 2003. Contraints for an interpretation of the italian geodynamics: a review. Memorie Descrittive della Carta Geologica d'Italia, LXII, 15-46.Selverstone, J., 1985. Petrological constraints on imbrication metamorphism and uplift in the SW Tauern Window, Eastern Alps. Tectonics, 4, 687-704.Selverstone, J. & Spear, F. S., 1985. Metamorphic P-T paths from pelitic schists and greenstones from the south-west Tauern Window. Journal of Metamorphic Geology, 3, 439-465.Selverstone, J., Spear, F. S., Franz, G. & Morteani, G., 1984. High-pressure metamorphism in SW Tauern Window, Austria: P-T paths from hornblende-kyanite-staurotide schists. Journal of Petrology, 25(4), 501-531.Spear, F. & Cheney, J., 1989. A petrogenetic grid for pelitic schists in the system SiO2-Al2O3-FeO-MgO-K2O-H2O. Contributions to Mineralogy and Petrology, 101(2), 149-164.Spear, F. & Selverstone, J., 1983. Quantitative P-T paths from zoned minerals: Theory and tectonic applications. Contributions To Mineralogy And Petrology, 83, 348-357.Spear, F. S., 1993. Metamorphic Phase Equilibria and Pressure-Temperature-Time Paths. Mineralogical Society of America, Washington, D. C.Staub, R., 1926. Geologische Karte des Avers. In: Beiträge zur geologischen Karte der Schweiz, Bern.Streckeisen, A., Wenk, E. & Frey, M., 1974. Steep isogradic surface in Simplon area. Contributions to Mineralogy and Petrology, 47(1), 81-95.Theye, T., Reinhardt, J., Goffé, B., Jolivet, L. & Brunet, C., 1997. Ferro- and magnesiocarpholite from the Monte Argentario (Italy): First evidence for high-pressure metamorphism of the metasedimentary Verrucano sequence, and significance for P-T path reconstruction. European Journal of Mineralogy, 9, 859-873.Theye, T., Seidel, E. & Vidal, O., 1992. Carpholite, sudoite and chloritoid in low-grade high-pressure metapelites from Crete and the Peloponnese, Greece. European Journal of Mineralogy, 4, 487-507.Todd, C. S. & Engi, M., 1997. Metamorphic field gradients in the Central Alps. Journal of Metamorphic Geology, 15, 513-530.Tribuzio, R. & Giacomini, F., 2002. Blueschist facies metamorphism of peralkaline rhyolites from the Tenda crystalline massif (northern Corsica): evidence for involvement in the Alpine subduction event? Journal of Metamorphic Geology, 20(5), 513-.Tribuzio, R. & Giacomini, F., 2002. Blueschist facies metamorphism of peralkaline rhyolites from the Tenda crystalline massif (northern Corsica): evidence for involvement in the Alpine subduction event? Journal of Metamorphic Geology, 20(5), 513-.Trommsdorff, V., 1966. Progressive Metamorphose kieseliger Karbonatgesteine in den Zentralalpen zwischen Bernina und Simplon. Schweizerische Mineralogische Und Petrographische Mitteilungen, 46, 431-460.Venturini, G., 1995. Geology, Geochemistry and Geochronology of the inner central Sesia Zone (Western Alps), Lausanne.Verdoya, M., Chiozzi, P. & Pasquale, V., 2001. Heat-producing radionucleides in metamorphic rocks of the Briançonnais-Piedmont Zone. Eclogae Geologicae Helvetiae, 94, 213-219.Vidal, O., Goffé, B. & Theye, T., 1992. Experimental study of the stability of sudoite and magnesiocarpholite and calculation of a new petrogenetic grid for the system FeO-MgO-Al2O3-SiO2-H2O. Journal of Metamorphic Geology, 10, 603-614.Vidal, O., Parra, T. & Trotet, F., 2001. A thermodynamic model for Fe-Mg aluminous chlorite using data from phase equilibrium experiments and natural pelitic assemblages in the 100-600°C, 1-25 kbar range. American Journal of Science, 301, 557-592.Vidal, O., Theye, T. & Chopin, C., 1994. Experimental study of chloritoid stability at high pressure and various fO2 conditions. Contributions to Mineralogy and Petrology, 118, 256-270.Wang, P. & Spear, F. S., 1991. A field and theoretical analysis of garnet+chlorite+chloritoid+biotite assemblages from the tri-state (MA, CT, NY) area, USA. Contributions to Mineralogy and Petrology, 106(2), 217-235.Wei, C. & Powell, R., 2004. Calculated Phase Relations in High-Pressure Metapelites in the System NKFMASH (Na2O-K2O-FeO-MgO-Al2O3-SiO2-H2O). Journal of Petrology, 45(1), 183-202.Wei, C. J. & Holland, T. J. B., 2003. Phase relations in high-pressure metapelites in the system KFMASH (K2O-FeO-MgO-Al2O3-SiO2-H2O) with application to natural rocks. Contributions to Mineralogy and Petrology, 301, 301-315.Wei, C. J., Powell, R. & Clarke, G. L., 2004. Calculated phase equilibria for low- and medium-pressure metapelites in the KFMASH and KMnFMASH systems. Journal of Metamorphic Geology, 22, 495-508.Wiederkehr, M., Bousquet, R., Ziemann, M. A., Schmid, S. M. & Berger, A., 2007. Thermal structure of the Valaisan and Ultra-Helvetic sedimentary units of the northern Lepontine dome - consequences regarding the tectono-metamorphic evolution. In: EGU, Wien.Yardley, B. W. D., 1989. An introduction to metamorphic petrology. Longman.Zeh, A., 2001. Inference of a detailed P-T path from P-T pseudosections using metapelitic rocks of variable composition from a single outcrop, Shackleton Range, Antarctica. Journal of Metamorphic Geology, 19(4), 329-350.Zimmermann, R., Hammerschmidt, K. & Franz, G., 1994. Eocene high pressure metamorphism in the Penninic units of the Tauern Window (Eastern Alps: evidence from 40Ar-39Ar dating and petrological investigations. Contributions to Mineralogy and Petrology, 117, 175-186.Zwart, H. J., 1973. Metamorphic map of Europe, scale 1:2'500'000. Subcommission of Cartography of the Metamorphic Belts of the World, Leiden / UNESCO, Paris.Zwart, H. J., Sobolev, V. S. & Niggli, E., 1978. Metamorphic map of Europe, scale 1:2'500'000. Explanatory text. Subcommission of Cartography of the Metamorphic Belts of the World, Leiden / UNESCO, Paris.